science
Overview: Rivers and estuaries play an important role in delivering land-derived material (e.g., sediment, contaminants) and freshwater to the coastal ocean, the latter impacting coastal circulation. Yet the role of near-shore waves and wave breaking in river plume mixing, transport, and ultimate fate along the coastline is not yet understood. This study will improve the dynamical understanding of small-moderate freshwater plumes (including river plumes, creeks, estuary outflows, and engineered structures) in the presence of breaking waves. Prior research suggests waves can influence plume behavior including enhanced along-shore spreading within the surf zone and decreased freshwater export to the inner-shelf, yet significant knowledge gaps remain in the understanding of wave/plume interactions across a range of plume discharge and across the surf zone/inner-shelf region. These knowledge gaps limit our ability to predict the ultimate fate of small buoyant plumes and the material they carry with them, relevant to coastal pollution, larval transport, and more. To address this knowledge gap, this NSF-sponsored project uses a combination of measurements and numerical models to determine the fate of buoyant river plume water in the coastal ocean.
PiNC observational campaign: One of the key observational components of this project is an experiment being conducted at Los Peñasquitos Lagoon (LPL), a small intermittently closed estuary in Southern California near the border between the cities of San Diego and Del Mar. 2-3 dye releases at the estuary mouth during January and early February 2023 will be used to track the outflowing buoyant plume across and along the surfzone. Fluorometers, jetski transects, and an unmanned aircraft system (UAS) will be used to capture the dye’s 3-dimensional structure and spatial extent. Oceanographic conditions (waves, currents, tides, salinity, temperature) will be monitored using in-situ sensors deployed for this project, in conjunction with long-term monitoring stations (e.g., NOAA, USGS, CDIP, NERR). The dye is an environmentally safe passive tracer and we have obtained all necessary permits to conduct this work.
Output from this observational campaign will be combined with a completed observational study in Washington, realistic numerical simulations of both field sites, and a suite of idealized numerical simulations to span parameter space. The improved understanding of river water mixing and fate in the coastal ocean that will result from this work is crucial to quantifying the spreading of sediment, pollutants, larvae, and other important material in the nearshore.
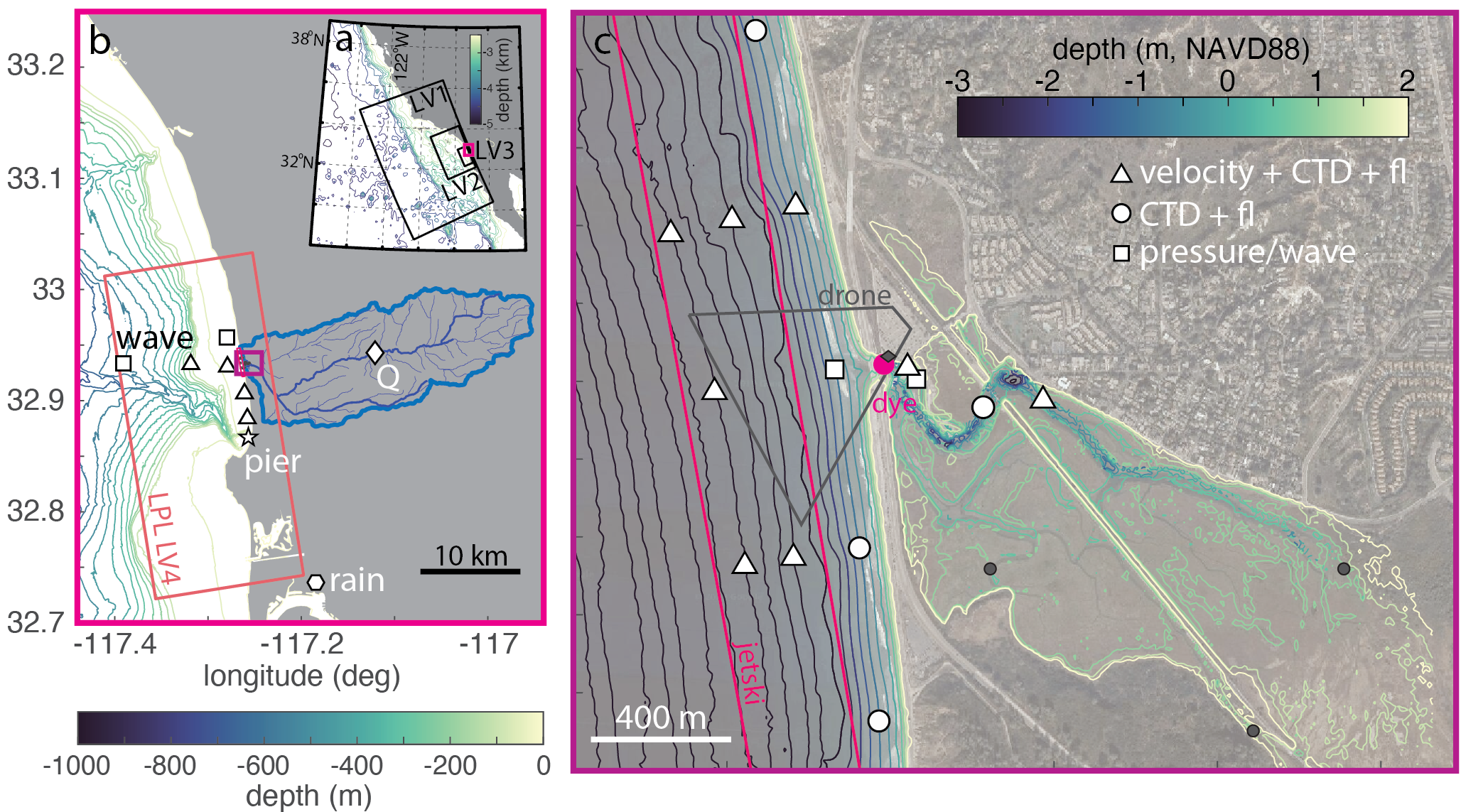
Hypotheses: The schematic to the right demonstrates the hypotheses we are testing with this work. Our first hypothesis (H1) is that the fresh river water will either be trapped in the surfzone (dashed line) OR may escape past the surfzone as a freshwater plume, or a combination of the two, and this behavior will be determined by the balance of wave and river forcings. Our second hypothesis (H2) is that we will be able to parameterize this system, and the likelihood of trapping vs escapes, in the context of other estuarine systems such as the Quinault and Maipo Rivers. If the freshwater is trapped in the surfzone, our third hypothesis (H3) is that the fresh water will primarily escape the surfzone through rip current activity. Last, we hypothesize (H4) that the freshwater will be mixed in the surfzone by waves, and that we will be able to model this using existing turbulence closure models.
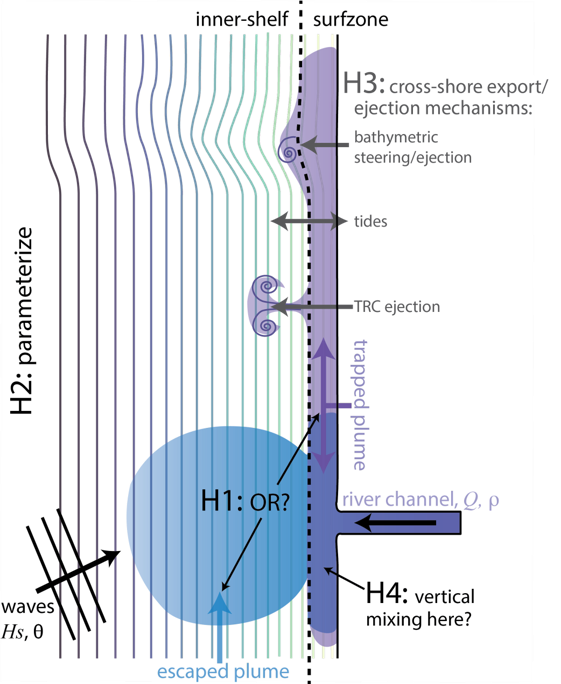
Prior research: Most prior plume studies have focused on individual large plumes; yet small-moderate discharge plumes may be of equal or even greater importance due to their combined fluxes (e.g., Mazzini et al., 2014, Morrison et al., 2012) and delivery of terrigenous material (e.g., Wetz et al., 2006). Such small-moderate discharge plumes may be dramatically modified as they transit the surf zone, where wave processes dominate mixing and circulation, and strongly affect the fate of plume water.
Many studies have investigated surf zone dynamics, including along- and across-shore tracer spreading from both beach-based (e.g., Hally-Rosendahl et al., 2014) and coastal discharge-based sources (e.g., Grant 2005; Wong et al., 2013; Feddersen et al., 2016). The latter have considered the momentum but not the buoyancy of the outlet. Many of these studies indicate along-shore surf zone currents (e.g., Longuet-Higgins, 1970) dominate transport. However, cross-shelf tracer transport is complex and often 3D, driven by a variety of processes, including surface gravity waves (through Stokes drift), fronts (Horner-Devine et al., 2017), and bathymetric and transient rip currents (TRCs). Bathymetrically controlled rip currents occur in channels or depressions that persist for days to weeks (MacMahan et al., 2006; Dalrymple et al., 2011), while TRCs are episodic and narrow, and often a primary driver of exchange between the surf zone and inner shelf (Spydell et al., 2007; Clark et al., 2010). The role of 3D TRCs in inner-shelf regions has only recently been identified (Kumar and Feddersen 2017a, b, c). Multiple studies highlight the importance of these surf zone transport processes on pollutant exposure (e.g., Boehm et al., 2005).
Several studies have investigated bouyant plume/wave interactions emphasizing an important role of depth-limited breaking waves on the ultimate plume transport. Using 2.1 m resolution aerial imagery Svejkovsky et al. (2010) found plume transport for a small discharge plume (<100 m3 s-1) correlates more strongly with wave direction than winds. Delpey et al. (2014) found that surf zone generated along-shore currents could overwhelm a very small (Q<5 m3 s-1) freshwater outflow into an embayment, keeping plume water along the embayment edges and reducing total freshwater flux into the bay in a realistic wave-current numerical model. A few recent studies have investigated the direct interaction of small coastal plumes with depth-limited breaking surface waves, all of which highlight the potential for waves to effectively trap plume water near shore and reduce the amount of freshwater exported beyond the surf zone. Idealized simulations (Rodriguez et al., 2018) and realistic simulations (Moghimi et al., 2019) highlight this trapping mechanism with the former providing a dynamical framework to examine the transition from plume trapping to plume export depending upon the competition of discharge momentum flux and wave momentum flux (see the figure below). Similarly, Wong et al. (2013) and Kastner et al. (2019) both describe observations with the same trapping phenomena. Wong et al. (2013) show that surf zone trapping of dye released in the mouth of a small creek emptying into the surf zone is determined by the degree to which the creek outflow is turned by wave-driven forcing while Kastner et al. (2019) use three length scales: the surf zone width, LSZ; the near-field plume length, LNF; and the cross-shore extent of the channel, LC to conceptualize the momentum balance. The relative length scales show how tidal variability and bathymetric effects change the balance of wave and river momentum. Based on observed discharge, waves, and tides, Kastner et al. (2019) predict that approximately 70% of the Quinault River discharge is trapped in the surf zone annually.
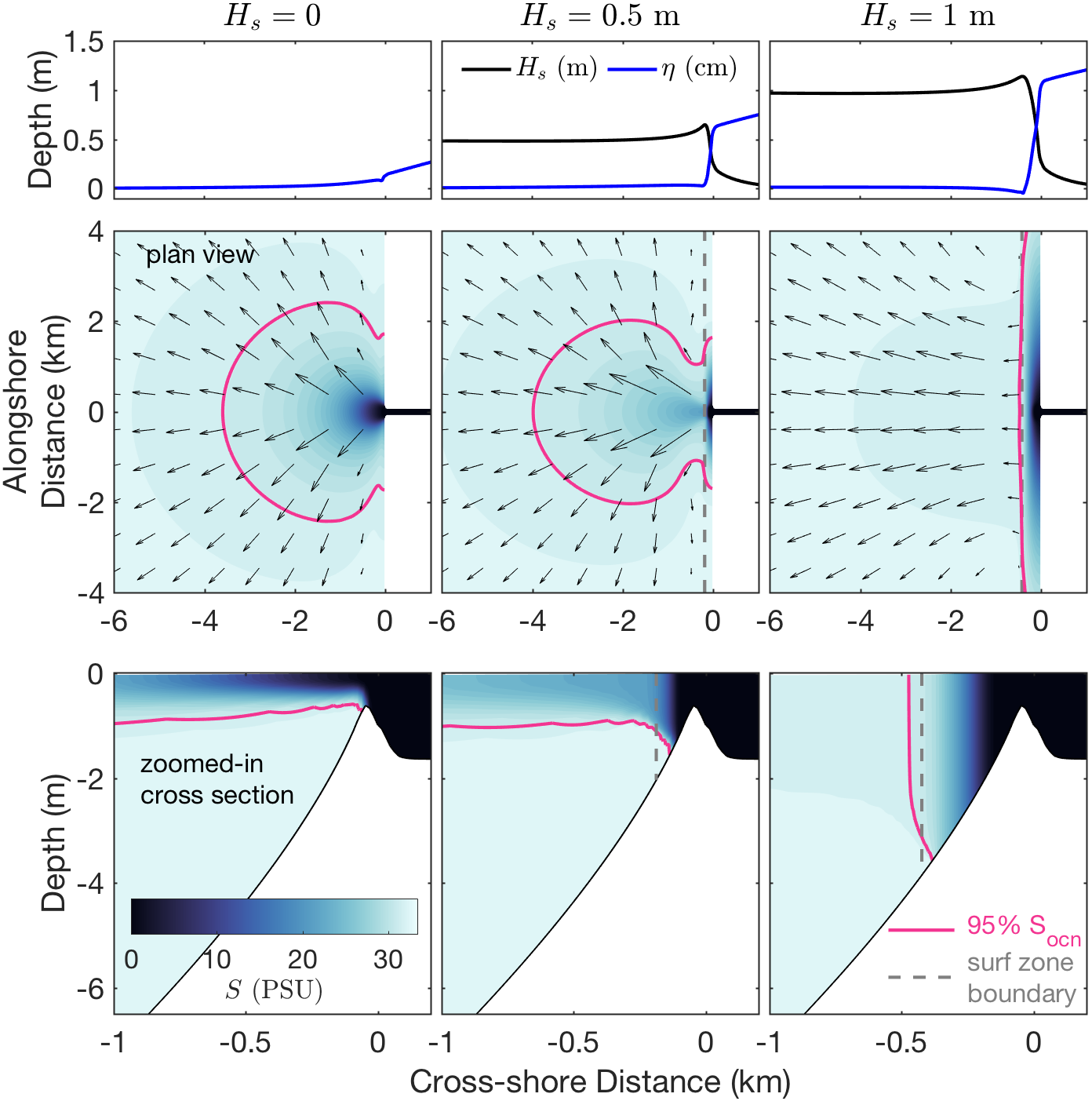
Idealized simulation results from Rodriguez et al. (2019). Model runs on an idealized grid showing hydrodynamic only (no waves, left) and fully coupled wave-hydrodynamic simulations with significant wave height, Hs = 0.5 m (middle) and Hs = 1 m (right), all with river flow, Q = 20 m3 s-1. Top panels show significant wave height (Hs, black) and sea surface height (η, blue) along the plume center. Middle panels show sea surface salinity and velocity vectors. Bottom panels show zoomed-in cross sections through the plume center. The pink line indicates the 95% salinity value while the dashed gray line indicates the surf zone boundary. For the same river discharge, the plume becomes more trapped and spreads further alongshore as the waves increase.
Ultimately, while prior research has shown important impacts of waves on plume structure and mixing at the plume front, gaps remain in our dynamical understanding of wave/plume interactions. In particular, we identified a significant gap in the current knowledge of wave impacts on small to moderate discharge plumes, and across the surfzone/inner-shelf region, the focus of this study. Recent idealized numerical studies and field measurements (described above) provide a rudimentary, yet incomplete understanding of these dynamics across the surf zone/inner-shelf regions and drive our hypotheses (described above). This project incorporating observations and modeling builds off of these prior studies, providing the first comprehensive study of the fate of river water in the surf zone that spans the full parameter space in which wave and plume processes interact in the nearshore.
References:
Boehm, A.B., D.P. Keymer, and G.G. Shellenbarger. 2005. “An Analytical Model of Enterococci Inactivation, Grazing, and Transport in the Surf Zone of a Marine Beach.” Water Res., 39 (15): 3565–78.
Clark, D.B., F. Feddersen, and R.T. Guza. 2010. “Cross-Shore Surfzone Tracer Dispersion in an Alongshore Current.” J. Geophys. Res., 115 (C10). doi: 10.1029/2009jc005683.
Dalrymple, R.A., J.H. MacMahan, A.J.H, and V. Nelko. 2011. “Rip Currents.” Annual Review of Fluid Mechanics, 43 (1): 551–81.
Delpey, M.T., F. Ardhuin, P. Otheguy, and A. Jouon. 2014. “Effects of Waves on Coastal Water Dispersion in a Small Estuarine Bay.” J. Geophys. Res., C: Oceans 119 (1): 70–86.
Feddersen, F., M. Olabarrieta, R.T. Guza, D. Winters, Britt Raubenheimer, and Steve Elgar. 2016. “Observations and Modeling of a Tidal Inlet Dye Tracer Plume.” J. Geophys. Res., C: Oceans 121 (10): 7819–44.
Grant, S.B., J.H. Kim, B.H. Jones, S.A. Jenkins, J. Wasyl, and C. Cudaback. 2005. “Surf Zone Entrainment, along-Shore Transport, and Human Health Implications of Pollution from Tidal Outlets.” J. Geophys. Res., 110 (C10). doi: 10.1029/2004jc002401.
Hally-Rosendahl, K., F. Feddersen, and R.T. Guza. 2014. “Cross-Shore Tracer Exchange between the Surfzone and Inner-Shelf.” J. Geophys. Res., C: Oceans 119 (7): 4367–88.
Horner-Devine, A.R., J.D. Pietrzak, A. Souza, S. Meirelles, M. Henriquez, M.A. McKeon, R. Flores, S. Rijnsburger, 2017. Cross-shore transport of surf-zone sediment by river plume frontal pumping. Geophys. Res. Lett., 44, doi: 10.1002/2017GL073378.
Kumar, N., and F. Feddersen. 2017a. “The Effect of Stokes Drift and Transient Rip Currents on the Inner Shelf. Part I: No Stratification.” J. Phys. Oceanogr., 47 (1): 227–41.
Kumar, N., and F. Feddersen. 2017b. “The Effect of Stokes Drift and Transient Rip Currents on the Inner Shelf. Part II: With Stratification.” J. Phys. Oceanogr., 47 (1): 243–60.
Kumar, N., and F. Feddersen. 2017c. “A New Offshore Transport Mechanism for Shoreline-Released Tracer Induced by Transient Rip Currents and Stratification.” Geophys. Res. Letters, 44 (6): 2843–51.
Longuet-Higgins,M.S.,1970,Longshore currents generated by obliquely incident sea waves:1,J. Geophys. Res., 75(33), 6778–6789, doi: 10.1029/JC075i033p06778.
MacMahan, J.H., E.B. Thornton, and A.J.H. 2006. “Rip Current Review.” Coastal Engineering 53 (2-3): 191–208.
Mazzini, P.L.F., J.A. Barth, R.K. Shearman, and A. Erofeev. 2014. “Buoyancy-Driven Coastal Currents off Oregon during Fall and Winter.” J. Phys. Oceanogr., 44 (11): 2854–76.
Moghimi, S., H.T. OĢzkan-Haller, C. Akan, and J.T. Jurisa. 2019. “Mechanistic Analysis of the Wave-Current Interaction in the Plume Region of a Partially Mixed Tidal Inlet.” Ocean Modelling 134: 110–26.
Morrison J,M. G.G. Foreman & D. Masson (2012) A Method for Estimating Monthly Freshwater Discharge Affecting British Columbia Coastal Waters, Atmosphere-Ocean, 50:1, 1-8, doi: 10.1080/07055900.2011.637667.
Spydell, M., F. Feddersen, R.T. Guza, and W.E. Schmidt. 2007. “Observing Surf-Zone Dispersion with Drifters.” J. Phys. Oceanogr., 37 (12): 2920–39.
Svejkovsky, J., N.P. Nezlin, N.M. Mustain, and J.B. Kum. 2010. “Tracking Stormwater Discharge Plumes and Water Quality of the Tijuana River with Multispectral Aerial Imagery.” Estuarine, Coastal and Shelf Science, 87 (3): 387–98.
Wetz M.S., B. Hales, Z. Chase, P.A. Wheeler, M. Whitney, 2006, Riverine input of macronutrients, iron, and organic matter to the coastal ocean off Oregon, U.S.A., during the winter, Limnology and Oceanography, 51, doi: 10.4319/lo.2006.51.5.2221.
Wong, S.H.C., S.G. Monismith, and A.B. Boehm. 2013. “Simple Estimate of Entrainment Rate of Pollutants from a Coastal Discharge into the Surf Zone.” Environmental Science & Technology, 47 (20): 11554–61.